By Mathieu Boudreau
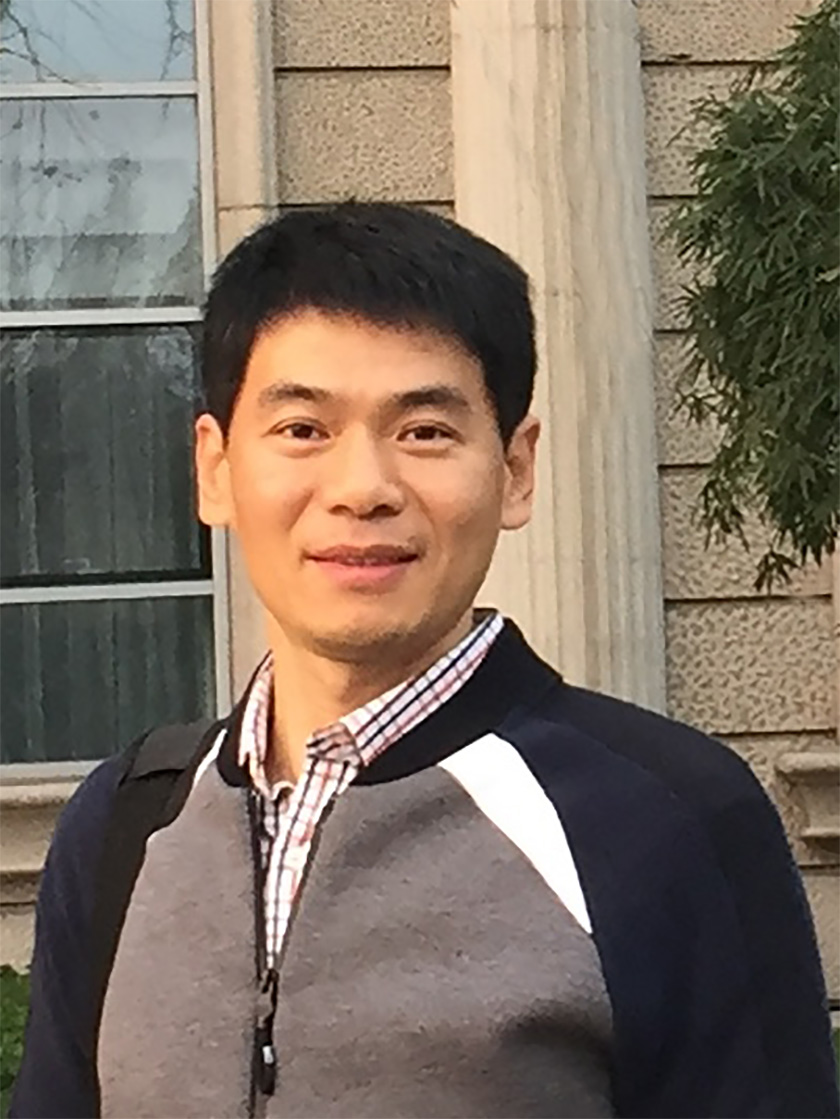
Xiao-Yong Zhang
The August 2017 Editor’s Pick is from Xiao-Yong Zhang and Zhongliang Zu, researchers at Vanderbilt University in Nashville. Their paper presents a newly discovered Nuclear Overhauser Effect (NOE) signal at -1.6 ppm from water. They measured this signal in normal rat brains at 9.4 T, and found that it changed significantly in a rodent tumor model. Using reconstituted phospholipids and cultured cell experiments, they hypothesize that this signal may originate from membrane choline phospholipids. We recently spoke with Xiao-Yong and Zhongliang Zu about their work.
Mathieu: Please introduce yourselves and tell us about your background.
Xiao-Yong: My background is in medical imaging. When I was doing my PhD in China, my research focused on MRI techniques. I then came to Emory University and Vanderbilt University for my postdoc, where I focused my research on MRI at the molecular level to detect brain diseases.
Zhongliang Zu: I got my PhD in medical physics at Peking University in China, and then came to Vanderbilt University for my postdoc, where I’m currently an assistant professor. My research focus is on MRI sequence development of chemical exchange saturation transfer (CEST), magnetization transfer (MT), and spin-locking techniques, and their applications in tumors, stroke, and other neurological diseases.
Mathieu: Before we dive into the paper, could you to explain briefly the MT and NOE mechanisms?
Xiao-Yong: Magnetization transfer (MT) is a physical process by which macromolecules and their closely associated water molecules cross-relax with protons in free water. And the Nuclear Overhauser Effect (NOE) is a nuclear spin-transfer phenomenon by a space-dependent dipolar coupling effect within molecules.
Mathieu: Can you give us an overview of your paper?
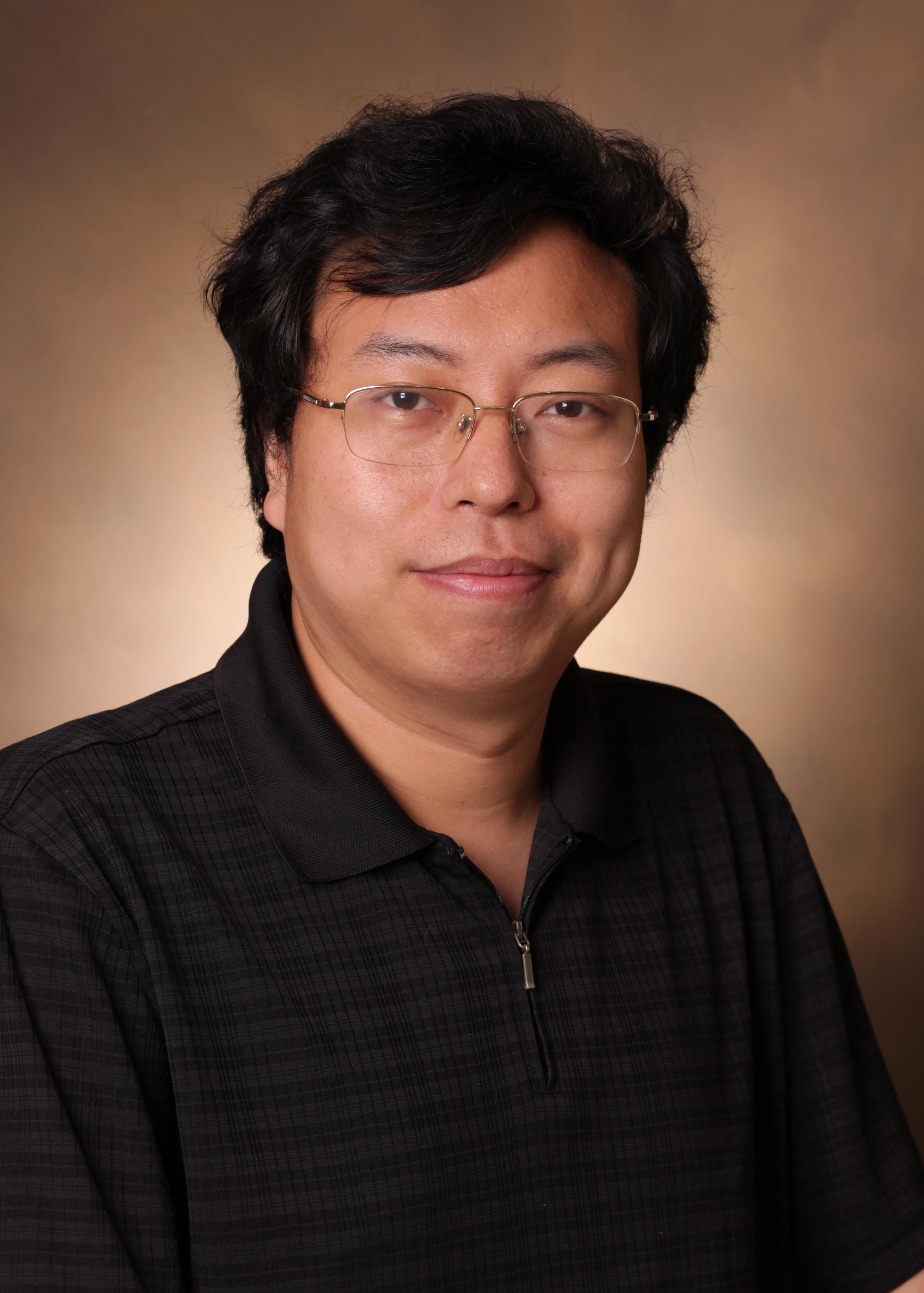
Zhongliang Zu
Zhongliang Zu: In this paper, we introduced how to quantify a newly discovered NOE signal at -1.6 ppm from water. Because it’s very close to the water peak, and thus is significantly influenced by direct water saturation effects, it’s not easy to directly observe on the CEST Z-spectrum. We used a quantification method to isolate this signal from other non-specific factors. We found that the amplitude, offset, and linewidth of this signal changed in some brain regions of healthy rats. We also explored the potential applications of this signal in a rat brain tumor model, finding that this signal changed significantly in tumors. Lastly, we used reconstituted phospholipids and cultured cell lines to study the possible molecular origin and contrast mechanism of this signal, and found that it could originate from membrane choline phospholipids and may be a new biomarker for diagnosing tumors.
Xiao-Yong: In my opinion, the origin of this signal is very important for us to know. The experiment on reconstituted phospholipids was just a preliminary study. For the next step, I think we should perform further studies on the origin of this signal. If we know the origin of this signal, it may open a lot of applications. It’s very interesting.
Mathieu: What were some of the technical challenges encountered during this work?
Xiao-Yong: One of the big challenges for this work was developing the quantification method, because this -1.6 ppm NOE signal is very broad and susceptible to direct saturation effect. It’s not as obvious as the typical -3.5 NOE signal, and they overlap. We tried several methods to quantify this signal. Finally, we used a fitting method to get our results.
Mathieu: Your quantification method corrects for T1 and B0. How sensitive is the signal to B1?
Zhongliang Zu: Correcting for B1 is a problem for the CEST community. For people working on clinical scanners, especially on 7T human scanners, where there is significant B1 inhomogeneity, they measure several Z-spectra with a series of powers, use a linear fit of the signal, and then correct for B1 effects. This may be applicable to our own method. But currently, we just performed the study on animal scanners, where B1 is not as much of a problem. If we further investigate our method on clinical scanners, we may try those correction techniques.
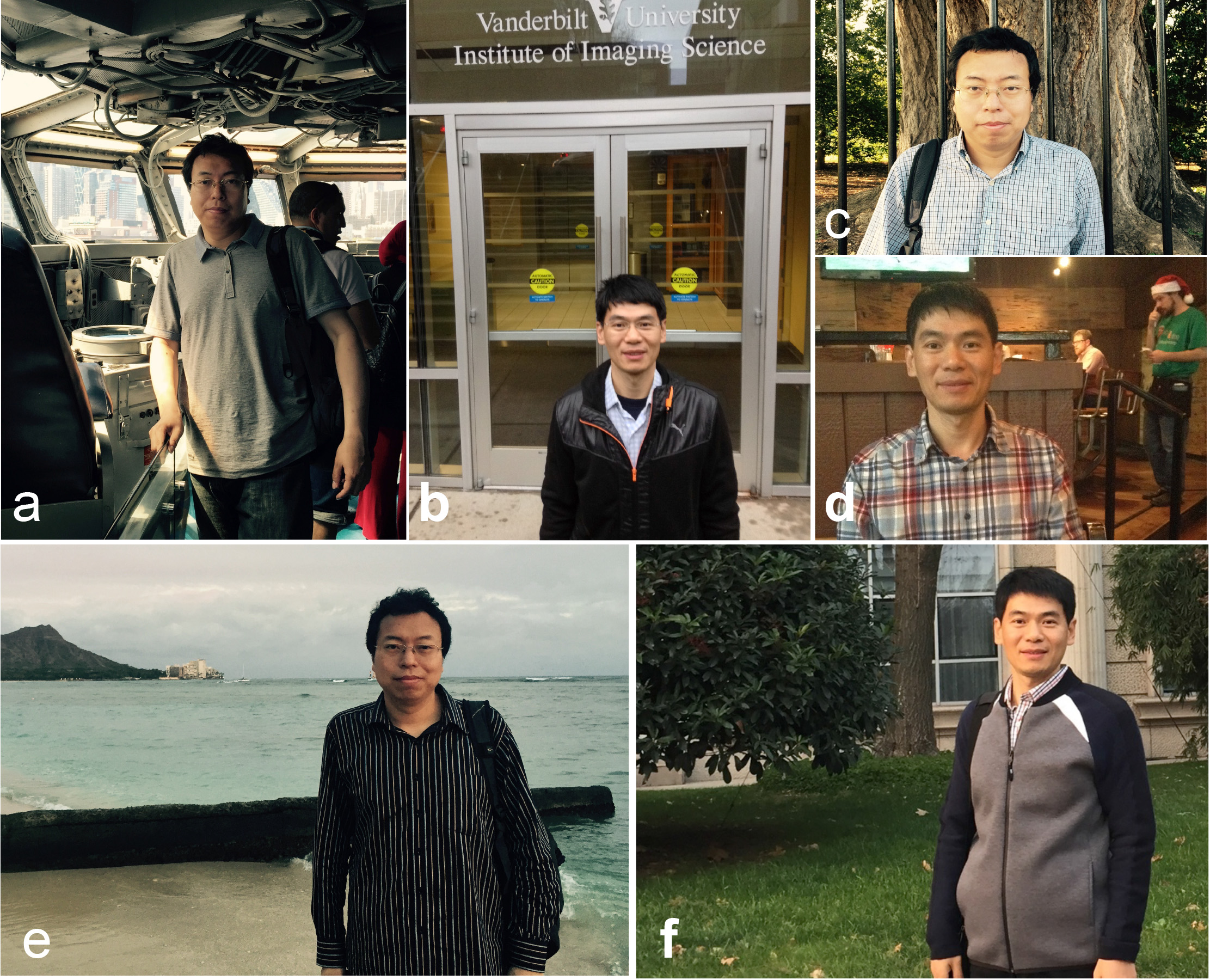
a) Zhongliang at the aircraft carrier USS Intrepid in New York; b) Xiao-Yong at Vanderbilt University Institute of Imaging Science; c) Zhongliang travelling; d) Xiao-Yong at a lab party; e) Zhongliang on the beach in Hawaii; f) Xiao-Yong on campus.
Mathieu: You mention in your paper that you noticed the -1.6 ppm NOE signal in other published work, but the authors didn’t report it. Do you see the need to revisit any other study that didn’t consider this effect?
Zhongliang Zu: Yes, definitely! I think the earliest paper where you can see the signal is one by Craig Jones at John Hopkins in 2013. In that paper, they studied NOE on humans at 7 T. There’s a figure in this paper where you can see a signal around -1.6 ppm. This signal can be found in healthy tissue, but not in tumors, which is in agreement with our study. They did not discuss this signal. There’s another paper by Kimberly Desmond in Toronto, from 2014. She showed that in mice the fitted error at -1 to -2 ppm is much bigger than at other offsets, which might mean there is something here.
Mathieu: What you do enjoy doing when you’re not in the lab?
Xiao-Yong: In my spare time, I enjoy playing ping-pong and Weiqi (Go).
Zhongliang Zu: During off-lab hours, I like to play some computer games, like Civilization and Ages of Empires [laughs]. Also, I’m in Tennessee, Nashville, which is a very beautiful place. I like to go to forests, and drive to nearby rural places.