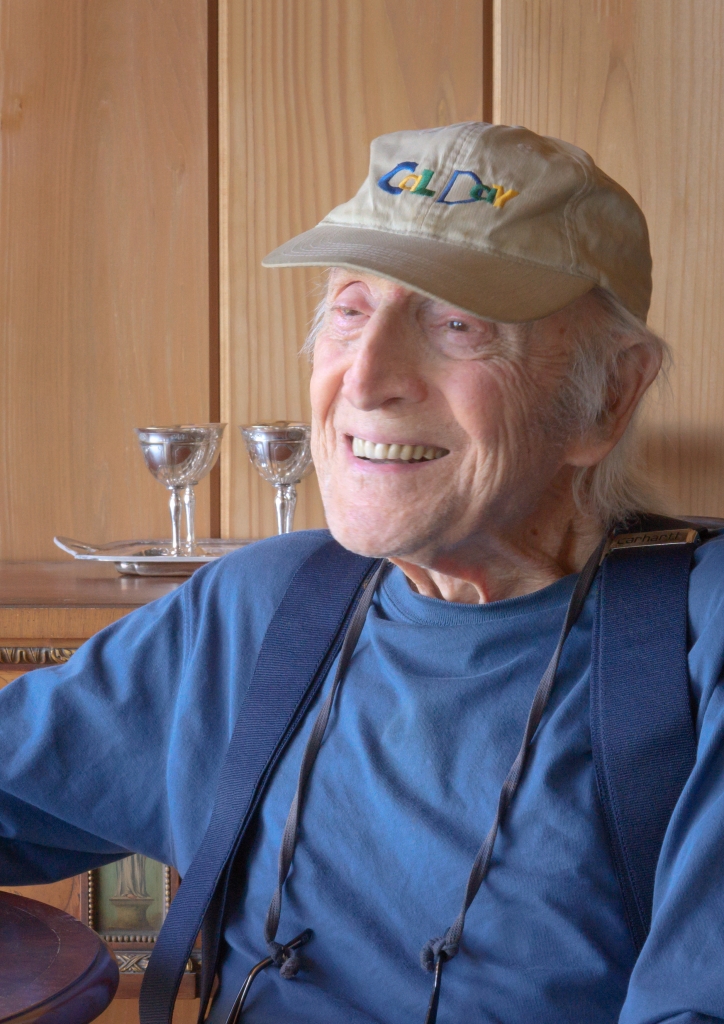
Erwin Hahn at his home in Berkeley, CA.
Download the digital version of our special Magnetic Resonance in Medicine Highlights magazine, including this interview with Erwin Hahn and more! (27MB PDF)
Interview was conducted by David Feinberg. Last summer I had the pleasure of meeting Berkeley professor emeritus, Dr. Erwin L. Hahn. His former (and final) graduate student, Larry Wald, was able to connect us in his hometown of Berkeley, CA. Over a hearty breakfast, Prof. Hahn had accepted my hopeful invitation to give the plenary talk at the upcoming ISMRM workshop on simultaneous multi-slice imaging. At one point, he asked me what I worked on in MRI and I replied “pulse sequence physics.” He then asked again, “Well, what do you do?” Only later did I realize the naiveté of my initial response.
In the days leading up to the workshop I spent many afternoons in his house, helping Prof. Hahn find and organize his slides for the plenary talk. It was there that I first saw in a slide (Fig. 1) his 1949 experiment to measure T1 by incrementally changing the timing between two RF pulses. I came to the realization that this was the very first pulse sequence! Erwin Hahn invented pulse sequences! Of course, I knew he discovered the spin echo, but I thought pulse sequences somehow came from the spectroscopy era, like babies from storks.
Pulse sequences are a specific time-dependent series of radio-frequency pulses and magnetic fields that produce MR signals, and are used to create essentially all imaging methods of MRI. Erwin Hahn is well-known for the discovery of the spin echo, but a fact often ignored by the MR community is that he was also the first to perform pulsed NMR (the first Free Induction Decay (FID)) and to describe the gradient echo. The FID was published in a brief Physical Review paper in 1949 (Phys. Rev. 76, 145), but was quickly overshadowed by the spin echo paper. The gradient echo was described in a 1960 paper on the MR detection of sea water motion, published in the Journal of Geophysical Research, and it is here that he also described bipolar gradient pulses to encode velocity phase shifts. Yet this and many of his contributions, no less his invention of the pulse sequence, seem to be obscured by history, lying in the shadow of the spin-echo.
Prior to Hahn’s research, magnetic resonance was performed by varying the main magnetic field H0 (now termed B0). This was done either by changing the field in a steady state search method as performed by Purcell’s group at Harvard, or by sweeping the H0 field through the resonance condition as performed by Bloch’s group at Stanford. Both of these techniques were performed in the presence of a continuously applied RF field, H1 (now termed B1), and henceforth called continuous wave (CW) techniques. Hahn’s transformative change was to perform magnetic resonance using a constant static B0 field without sweeping or varying this field, and applying a pulsed B1 at the Larmor resonance frequency, as now performed by modern day MRI.
It has been thrilling to talk with Erwin Hahn and to learn about what is essentially the creation of modern magnetic resonance. The following interview is an attempt to shine a light on his invention of the pulse sequence, as well as the design of his magnetic resonance instrument.
DF: After the war you were in a high energy physics group?
EH: When I came back from the war, I was working under Donald Kerst who invented the Betatron which was used to study gamma rays, and later used for medical purposes. And I was unhappy with it because I was just building power supplies, and not learning anything new. Then a theoretical physicist named James Bartlett pointed out papers
DF: You were a radar instructor during the war?
EH: In radar they use X band [8-12 GHz] and they would beat two microwave frequencies together, to get an intermediate frequency of 30 MHz, like in radio, only it’s wideband, unlike the radio, and could excite a larger number of nuclei. We had experience with that, so we set it up. Then we used a multivibrator to make square waves and synchronize.
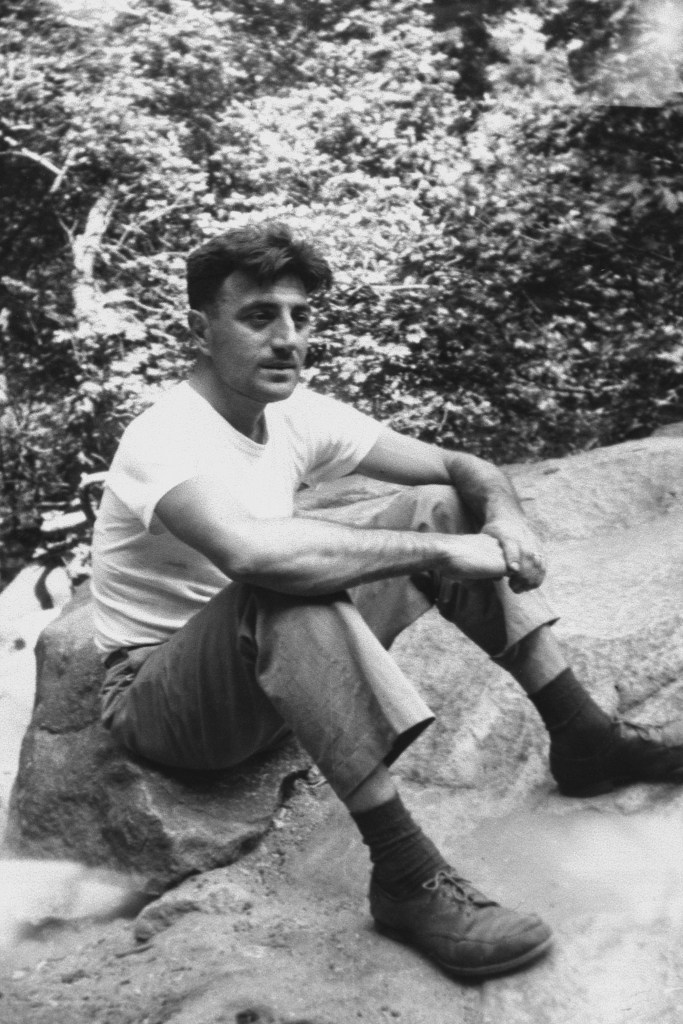
Young Erwin Hahn sitting on Lambert Dome rock in Yosemite Park, from Erwin Hahn’s scrapbook. Courtesy of Lawrence Berkeley National Laboratory.
DF: What motivated you to use the multivibrator pulse counter?
EH: It’s called a Higginbotham counter. It came from Los Alamos, and they possibly pulsed it for bomb systems. Some details of the atomic bomb research came out quite early after the war. I think information was informally available through gossip, and some was published shortly after the war was over, in a little magazine, a little booklet that was very valuable. And I got help from a lot of people in terms of information, and together with a good technician we set up the instrument (Fig. 2, 3). I put it in because I saw it could make things much easier. This was after my thesis, when I rebuilt my apparatus. I was a post doc when I did it. I started it before I got my degree, it was in the back of my mind that it could be done, but I had to finish my topic, which was on Rabi flops.
DF: The multivibrator is interesting given its convenience for pulse sequence timing.
EH: Well, I wanted to measure relaxation times and how they changed with time during a [chemical] reaction. In order to do that, I wanted to measure intervals of the decay curves. I wanted to measure the abscissa of growth or decay of T1 or T2, not to see the whole thing, but to see pieces. So after my thesis I stayed on and I developed the capability of applying RF pulses, namely leaving a gap between the pulses, whereas Bloch left the RF on all the time. Everybody did. However with the multivibrator system I could do the experiment by turning it on and governing where it would cross the sine wave, and know what the distance was in time, and therefore I could build up a superposition of signals on the Land camera [instant camera, precursor to the Polaroid, used to image the oscilloscopes’ output], by many repetitions of the experiment (Fig. 4).
DF: But what was your intention for doing this?
EH: Well at that time, I was changing the time between the pulses to get a very accurate measure of abscissa. I wanted to measure chemical reactions, by looking at T1 and T2 of the reactants as the reaction proceeded. I was a chemistry major in undergraduate school. That’s what interested me and I knew about rate equations and also about tuned circuits as I had done tuned circuitry in the navy. So I combined all these things.
DF: I’m interested in your instrument compared to Purcell lab’s instrument.
EH: They didn’t have RF pulses, nobody else was pulsing, not the way I was pulsing. No, well radar has pulses, the closest thing, and pulses have been used in submarines, in battleships and what not. But for this it was a different application. Radar was my business, because I taught it during the war. But anyone could have done it.
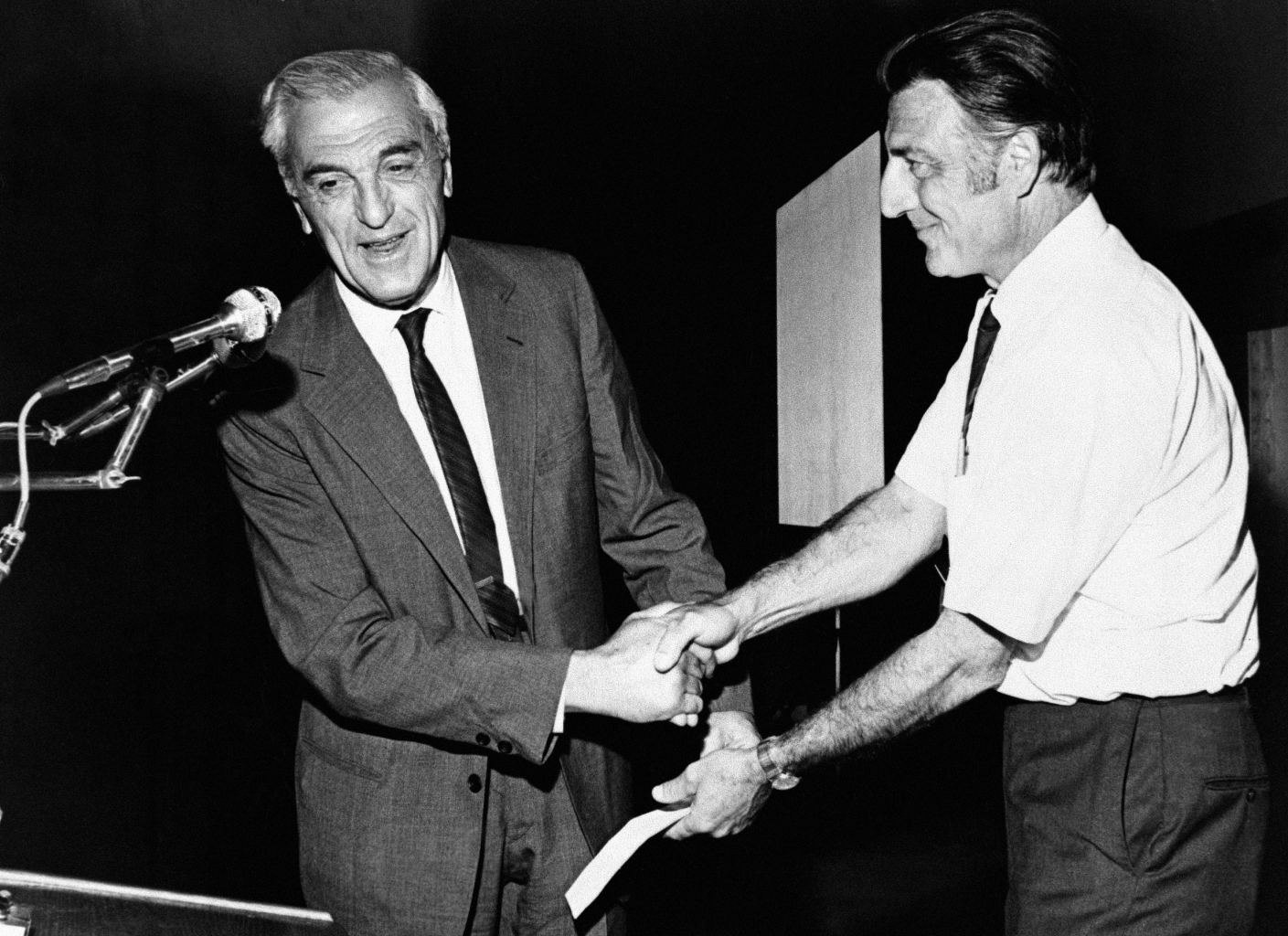
Felix Bloch shaking hands with Erwin Hahn. Courtesy of the Lawrence Berkeley National Laboratory.
DF: I know Torrey was doing similar work, but did he have the ability to change the timing?
EH: No, well Torrey turned on a step function of magnetic field and he would tune onto resonance. And then as soon as he tuned on, he got a decay. He was measuring Rabi flops like I was, unknowingly. He would turn H0 on for a certain interval and then turn off the H0 field at certain intervals.
DF: He wasn’t using two pulses?
EH: No, he was changing the application of the H0 field on resonance. In other words, it would precess as long as the field was on, but as soon as he shut the field off he got nothing moving off resonance. So he could not get an echo or FID being way off resonance. All he did was turn the DC field on and off.
DF: Did you know of his work?
EH: No, I didn’t know anything about it. In my PhD thesis I talked about nutations, called Rabi flop oscillations, but Torrey did the same experiment, he beat me to the punch by publishing it first. Although my thesis was Rabi flops, Charlie Slichter misinterpreted it. He kept telling people that I discovered echoes as a graduate student, but I discovered echoes afterwards. In my 1949 paper, I talked only of the FID and said I would talk about the echo next.
DF: Your 1949 paper was the first description of a pulse sequence?
EH: That’s right.
DF: A colleague asked me to ask you what made you most excited in your work?
EH: When I discovered the echo.
DF: At what point did you feel you really had something?
EH: When I found out that so many variables were dependent on the echo and exposed. I saw beats, I saw not only exponential decay but other effects, diffusion, chemical shift, cross-coupling, J-coupling. I got a couple of these effects right away. I was exhilarated.
DF: Was it all in a day or a night or a week?
EH: A week. I happened to narrow the RF pulse and by accident I got this thing and I said hey, what is going on? What’s that thing on the right, and I said there is something wrong with the apparatus! I kicked it and it went away. I narrowed the pulse more, I got a bigger echo! That’s when I realized I had something. The first thing I saw was an echo, and then an FID for the first time. The FID didn’t show up first, the echo showed up first. Then I applied the pulse again at a known time (Fig. 5, top). At an equal time later I saw a spin echo crossing the zero line at an equal number of cycles of the bias field Δω (3 Gauss at 60 cycle). The spin echo occurred at exactly the same point where the field is that of the magnet. Then on either side is like a Bloch sweep – down to up and up to down.
I realized that an FID was being produced, but Δω was taking it off-resonance. I shut off the sweeping bias field and it was beautiful (Fig.4). I didn’t need it. I just kept the DC field stable by regulating the current of H0 better. The bias field was turned off after the discovery of the spin echo (Fig. 5 bottom). I didn’t need it if the H0 was stable enough. I also realized the RF pulses needed more power. I had to adjust both the current of the main field and adjust the H1. Actually I was doing radical variations of at least four parameters. I maximized everything. I was pioneering the use of the sweep to go through resonance, but I found it by moving the Δω to the zero crossing, when the bias field is zero and I could turn off the bias field as the H0 was on resonance.
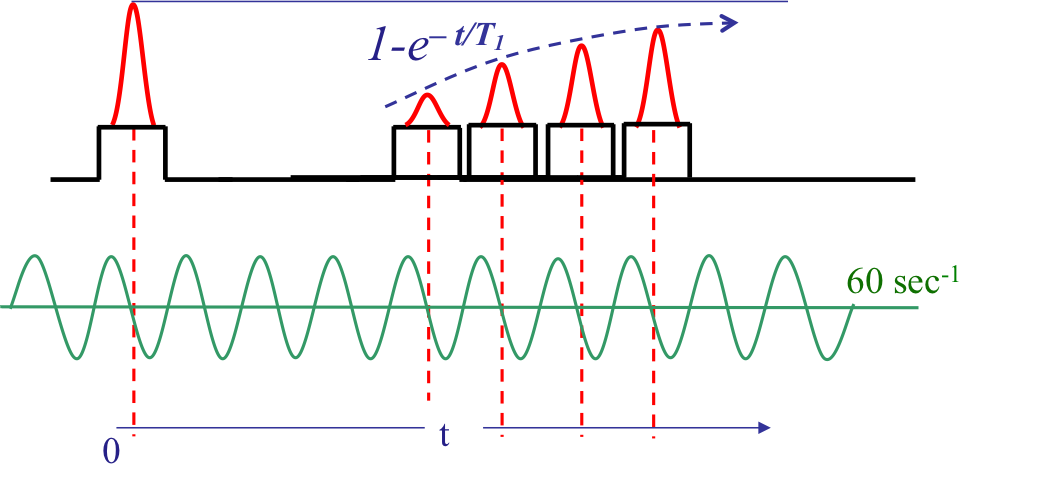
Figure 1. The first pulse sequence. To measure T1, the multivibrator incrementally changed time between the first H1 pulse and second H1 pulse shown at different time delays (Hahn, Phys. Rev. 76, 145 (1949)). Adaptation of Bloch adiabatic fast passage provided by Erwin L. Hahn.
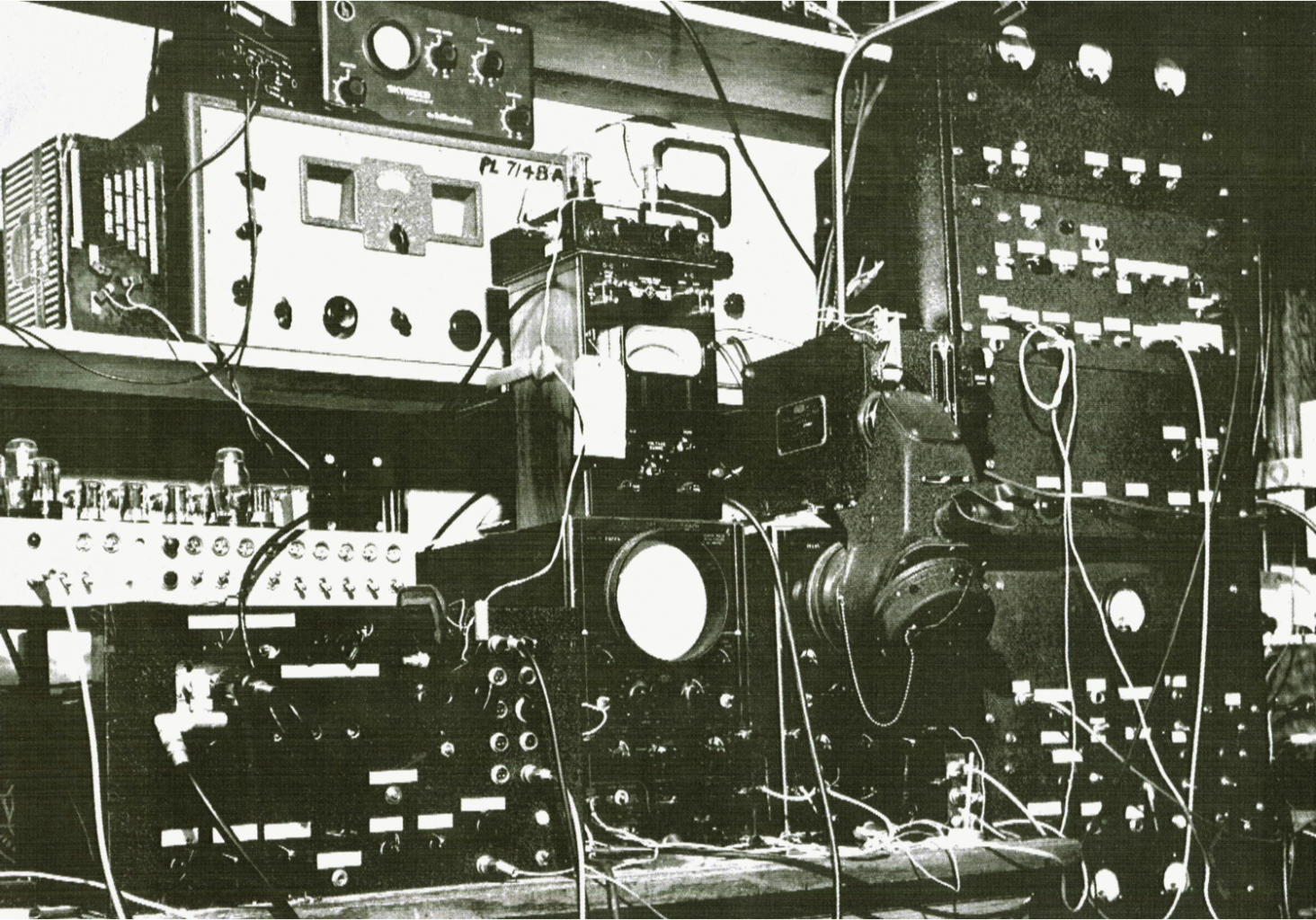
Figure 2. The apparatus on which the spin echo was discovered. Left center: Higginbotham multivibrator pulse generator and timer. Lower center: Land camera.
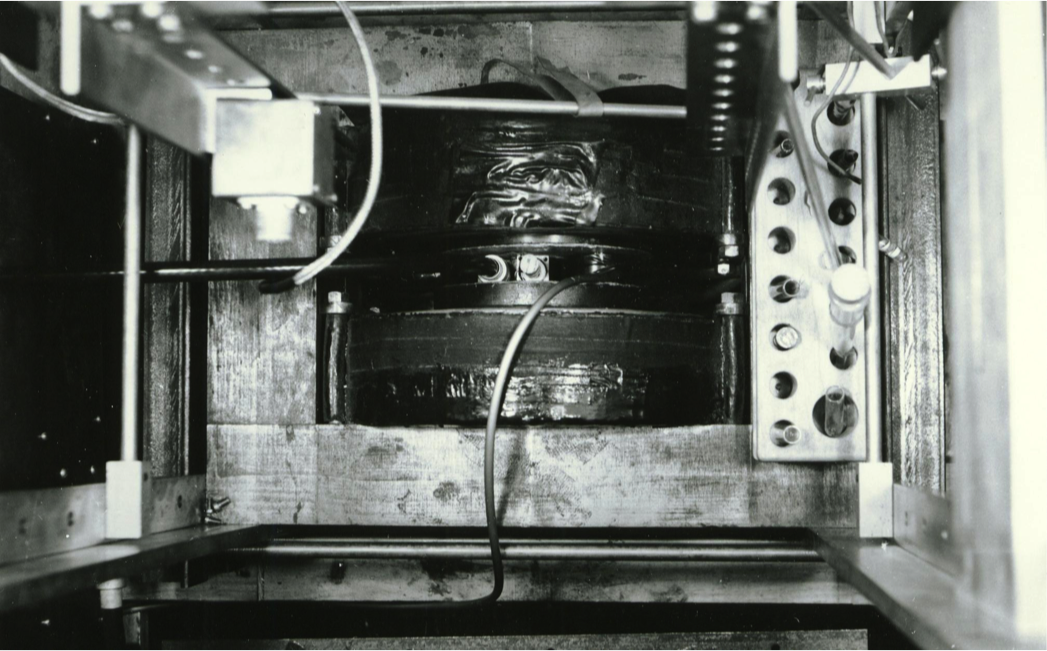
Figure 3. The magnet that Hahn “swiped” from a cyclotron and used for his magnetic resonance apparatus.
DF: How did things go after you first saw the echo?
EH: Actually, the echo went away for a week. All my parameters were off. I had it for one day. I thought perhaps the multivibrator was misfiring. A glitch. And then it came back and I stopped fiddling and I started varying parameters – change B and then change A parameter, etc, and I found I could start to optimize this funny signal. I turned off the oscillating bias field when I tuned the system to the Larmor resonance frequency. This helped stabilize things. It was a high current Varian magnet and difficult to control with low current. I breathed a sigh of relief because I was on the verge of giving up and would have missed the whole discovery, and it would have been easy to miss. I thought at first it could be a rogue wave, but I went to the Bloch equations and found the significance, that varying the amplitude of H0 or H1 does the same thing mathematically. I once made a narcissistic remark, I said, “Why didn’t these guys do it first, they’re supposed to be the experts.” I just varied as many parameters as I could and fought instabilities and incompetencies of circuit designs, and kept careful record to get through a jungle of blindness.
DF: Did you go around and talk to people about it?
EH: Yes. Arnold Nordsieck was a professor at Illinois, and he was on my thesis committee. He was a theoretical physicist, and magnetic resonance had just come along, so I showed him the work. Two days later, while he was working on a lathe, he looked at it, and said that’s interesting, and went back to his lathe. He wasn’t interested. He was building an analog computer. He was so busy with his amp.
DF: Early days in MRI must have been exciting?
EH: It was Lauterbur who was getting all the recognition. But it was Mansfield who was bringing up the rear. Lauterbur stuck with the back projection and I remember when he was lecturing in Washington from the stage, turning and looking at me, “And Hahn, you don’t need to use pulses.” Then up came Mansfield, with the echo-planar imaging with phase and frequency encoding. In fact I must say I heard that Mansfield put me up for the [Nobel] Prize several times, but not Lauterbur.
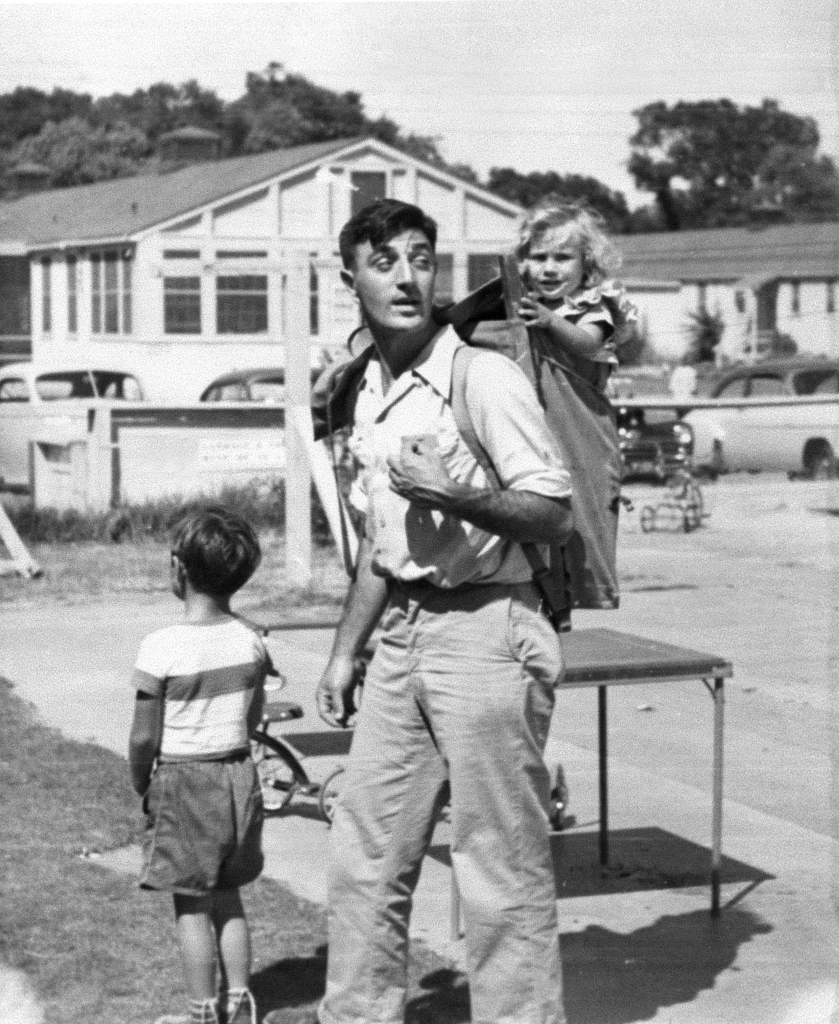
Erwin Hahn with his children, from Erwin Hahn’s scrapbook. Courtesy of Lawerence Berkeley National Laboratory.
DF: How about the gradient echo?
EH: I described it, and Bob Pound suggested I publish it. People got hold of it and expanded it. Anyway, that’s how it went.
DF: (referring to Hahn, J. Geophysical Res. 65, 776 (1960)) It’s interesting how you completely described changing the current direction in the gradient coil, and the signal would be maximum when the two gradient pulse time durations were the same.
EH: That’s right, it’s a forward backward race all over again. It’s refocused in the sense that it keeps going in the same direction but you change the phase, and it’s all generally the same thing. Just because you twist something it’s a new invention? I think one begets the other; it’s in the same kind. I didn’t pay much attention to it as I realized it was obvious. It’s obvious! I called it the Bloch method because he swept through.
Now the difference between Purcell and Bloch is that Purcell looked at absorption, they looked at a meter and they looked at the Q of the coil. Bloch looked at the signal dynamically. Purcell used the standard optical way of looking at photon absorption, but Bloch’s method turned out to be equivalent. There really was a big fight going on. In the beginning the two camps said – what are we doing here? You are doing something different. They finally came to an agreement that they were doing the same thing.
DF: How did other work affect your thinking?
EH: Rabi sensed there was a resonance, as I remember once Ramsey, his graduate student, said in an informal talk. Bloembergen noted that Julian Schwinger (who got the Nobel prize together with Feynman) did the quantum mechanics theory that explained what a step function did, verifying Bloch’s dynamical equations. It was Schwinger’s equation that I recalled when I first used pulses. I got an important hint on his equation from Bloembergen’s thesis, it was a great stimulus to me. Please quote me on this. Bloembergen won the Nobel prize later, really for the laser instead, but I’ve acknowledged him several times.
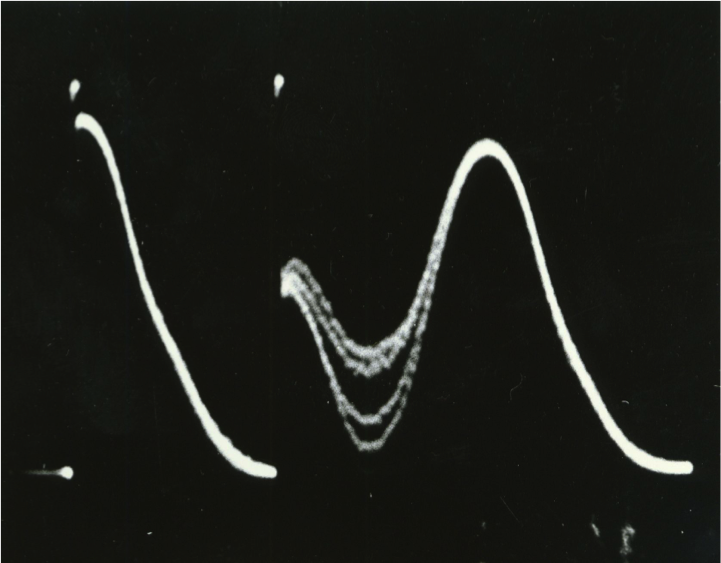
Figure 4. Spin echo and FID signals. The second RF pulse was not phase-correlated with the first RF pulse causing beating in the second FID (seen as 4 different height curved traces in repeated exposures super-positioned on the Land camera). The spin echo (far right) is unchanged. Provided by Erwin Hahn.
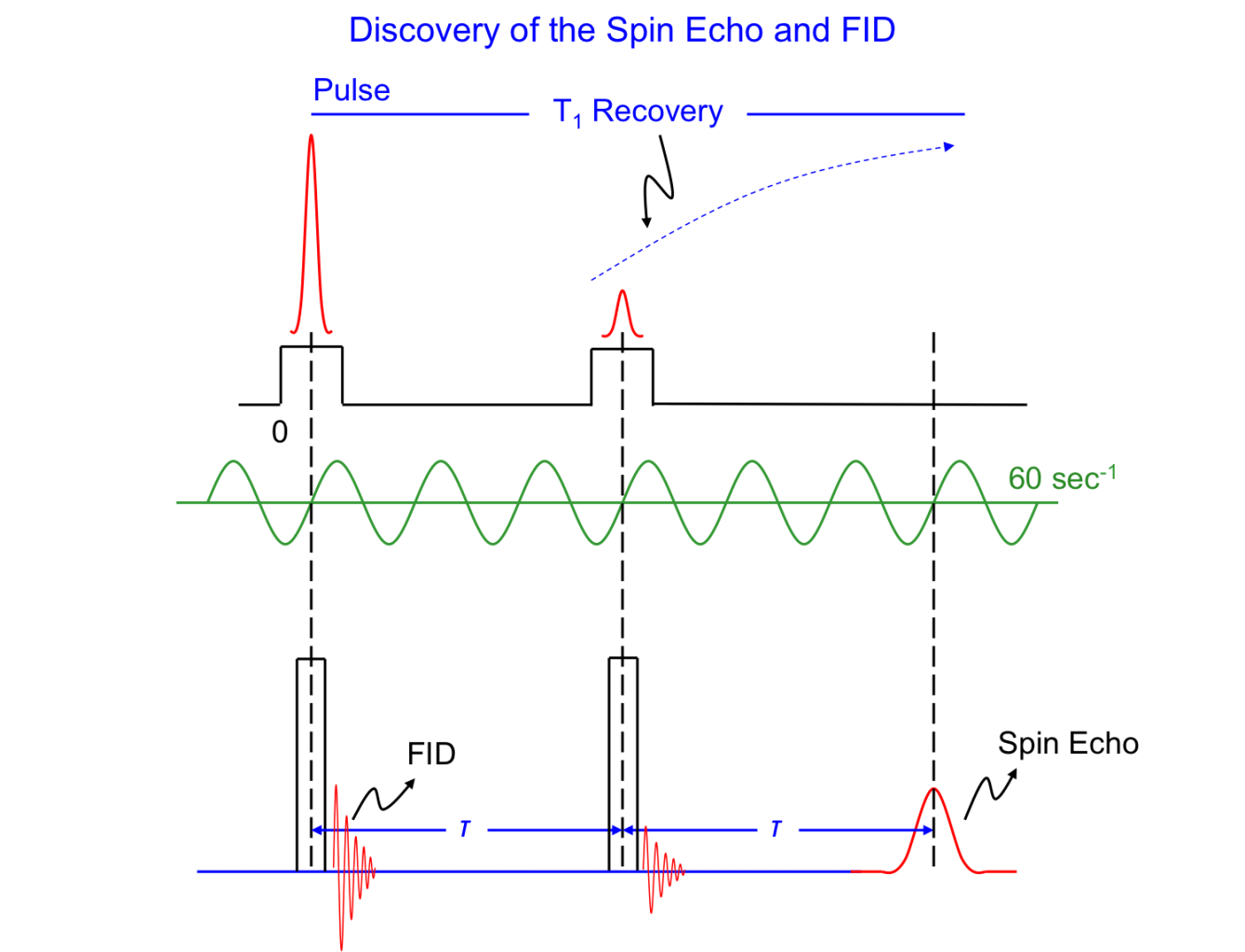
Figure 5. Top: Pulse sequence used to discover the Spin Echo. Bottom: Optimized pulse se¬quence. Tuning to Larmor resonance allowed for turning off the sweeping bias field. The higher H1 pulse power produced stronger spin echo and FID signals. Provided by Erwin Hahn.
DF: You combined your knowledge of pulses in radar with magnetic resonance, and you did this before spectroscopy and before MRI. MRI is inherently an application of pulse sequences, and it could not exist without echoes. That’s because of your inventions, not because of spectroscopy.
EH: Spectroscopists spent a lot of time publicizing what they did, and publishing on it over the years, and I didn’t. This didn’t put me in the limelight. It put them in the limelight. That’s what happened.
DF: But that doesn’t matter because your contributions to MRI are the introduction of echoes and pulse sequences. One can’t change history, I only want to remind and educate MRI scientists of your work.
EH: Well that’s very fine, that’s lovely, but it’s too late.
DF: It’s not too late for people to know where the fundamental innovations came from.
EH: No well that’s fine.
DF: Each year, MRI continues to become more vital to medicine and science, so it is important to know where the transformative work creating the entire field of MR came from. It seems to many people to be a great injustice that Erwin Hahn has not received, or at least shared the Nobel Prize in the many times it has been given for discoveries in magnetic resonance. Peter Mansfield wrote in the Epilogue of his 2013 autobiography, “I can say categorically that without Erwin Hahn’s contribution to the principles of spin echoes, there would be no MRI today… his contributions were and remain the cornerstone to the whole concept and implementation of MRI as it is used, not only in the ultra-high speed imaging of the type with which I have been personally connected, but also with the many general aspects of MRI as they have evolved and as they currently exist today.”
Last year Richard Ernst wrote to me in an email, “For me it is clear that an ISMRM medal for Erwin is too small a prize for him. Surely, He deserves the Nobel Prize!! And I have tried it more than 10 times without success so far. May be this or next year!”
David Feinberg works in the field of MRI pulse sequences for fast imaging, velocity and diffusion measurements. He led the optimization phase in the Human Connectome Project creating pulse sequence and gradient hardware advances for diffusion and fMRI. Several pulse sequences he innovated are now in general use; inner volume (zoomed) imaging, partial Fourier imaging, Twice refocused SE diffusion, gradient and spin echo (GRASE) and EPI variants including fly-back EPI, multiplexed EPI, and different simultaneous multi-slice techniques. At the start of his career, he published the earliest MR images of blood velocity in human vasculature, and of CSF velocity and brain motion. He was the first chair of the ISMRM Study Group on Quantitative Flow and Motion that standardized velocity phase imaging. He is the primary inventor of ASL 3D GRASE, which is becoming popular for clinical applications. His current research as principle investigator of a BRAIN Initiative project is to design a very high resolution MRI scanner for human neurosciences. He is a Fellow of ISMRM, president of Advanced MRI Technologies, and a professor at U.C. Berkeley.